In today’s world, sustainability is no longer just a buzzword—it’s a critical component of how we design, build, and operate buildings. Leadership in Energy and Environmental Design (LEED) has emerged as the global standard for green building certification, driving efforts to reduce environmental impact, enhance energy efficiency, and create healthier spaces for people.
Whether you’re seeking to certify a building or looking to establish yourself as a sustainability expert by earning a LEED credential, understanding the benefits and pathways is essential. This article explores what it takes to achieve LEED certification for buildings and individuals, diving into the levels of certification, rating systems, and professional credentials that empower you to lead the charge toward a greener future.
If you prefer to watch the video of this presentation, then scroll to the bottom.
LEED certification requires meeting certain standards and benchmarks that reduce the environmental impact of a building. Here’s how the process typically works:
LEED Certification Requirements for Buildings
1. Registration:
A project must first be registered with the Green Business Certification Inc. (GBCI) to start its LEED journey.
2. Select Rating System:
LEED has different rating systems based on the building’s function (e.g., healthcare, schools, homes). Selecting the appropriate rating system aligns the project’s goals with LEED’s performance expectations.
3. Meet Prerequisites and Earn Points:
Each LEED category has prerequisites that must be met. Beyond that, projects earn points in various credit categories, contributing toward the final certification score.
4. Submit for Review:
Upon completion, a project submits its documentation to GBCI, which reviews and determines the certification level.
LEED Certification Point Scale
LEED certification is awarded based on a points system, with each level signifying a higher standard of sustainability:
- Certified: 40–49 points
- Silver: 50–59 points
- Gold: 60–79 points
- Platinum: 80+ points
Each level represents an incremental achievement in sustainability practices, with Platinum being the pinnacle of resource efficiency and sustainability.
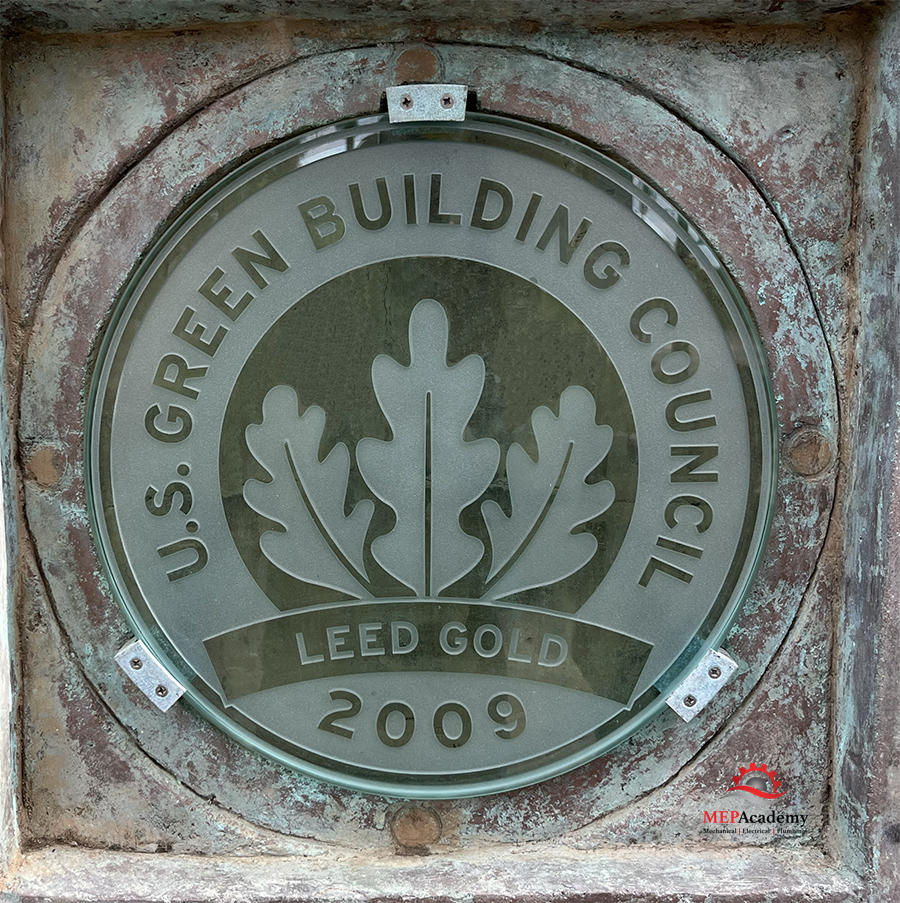
LEED Certification Types
LEED certification is versatile and applies to various building types. Each building type follows specific rating systems designed to measure unique environmental challenges. Here are the major LEED rating systems:
Building Design and Construction (BD+C):
For newly constructed or renovated buildings, covering commercial buildings, schools, retail, healthcare, data centers, hospitality, and warehouses.
Interior Design and Construction (ID+C):
Focuses on the interior fit-out of buildings, often used for retail and commercial interiors.
Building Operations and Maintenance (O+M):
Targets existing buildings, aiming to enhance operational efficiency and reduce environmental impact without major construction changes.
Neighborhood Development (ND):
Designed for residential and mixed-use communities, emphasizing walkability, connectivity, and green infrastructure.
Homes:
Intended for single-family or multifamily residential buildings, focusing on resource efficiency, indoor environmental quality, and energy savings.
Cities:
LEED for Cities provides a framework for entire cities or specific urban districts to measure and manage key sustainability metrics. These projects focus on tracking and improving water consumption, energy use, waste management, transportation systems, and the overall human experience within the urban environment.
LEED Points System
Each rating system is organized into specific categories, with points awarded based on performance across these aspects. LEED focuses on the following main categories:
Sustainable Sites (SS): Recognizes buildings that minimize their impact on ecosystems and water resources.
Water Efficiency (WE): Aims to reduce indoor, outdoor, and process water use through efficient fixtures, landscaping, and conservation.
Energy and Atmosphere (EA): Encourages optimization of energy performance, with a focus on energy use reduction, renewable energy, and ongoing monitoring.
Materials and Resources (MR): Promotes responsible material sourcing, recycling, and reducing waste generated by building occupants.
Indoor Environmental Quality (IEQ): Focuses on air quality, lighting, acoustic comfort, and the use of low-emitting materials to enhance occupant well-being.
Innovation (IN): Recognizes creative, sustainable design and operations practices that go beyond standard requirements.
Regional Priority (RP): Awards extra points for addressing local environmental issues, such as conserving regional water supplies or air quality.
LEED-certified buildings not only reduce operational costs but also attract environmentally conscious tenants, demonstrate commitment to sustainability, and support employee health and productivity. Moreover, many jurisdictions offer tax incentives, grants, or zoning benefits to promote sustainable construction practices.
LEED Professionals
Earning a LEED professional credential by passing the certification exam offers numerous advantages for individuals in the construction, design, and sustainability fields:
Enhanced Career Opportunities: LEED accreditation is recognized globally, making you a valuable asset to employers seeking expertise in green building practices.
Achieving Sustainability through LEED Certification and Industry Recognition: LEED credentials demonstrate your knowledge of sustainable building principles and your commitment to environmental stewardship.
Increased Marketability: As sustainable practices become a priority, having LEED credentials positions you as a leader in the field and differentiates you in a competitive job market.
Professional Growth: Learning about LEED systems and sustainability principles helps you stay current with the latest trends, technologies, and regulatory requirements in the industry.
Networking and Community: Becoming certified connects you with a network of sustainability professionals, offering collaboration and growth opportunities.
Contributing to a Better Future: By supporting green building practices, you play a key role in reducing the environmental impact of the construction industry.
LEED Professional Credentials
LEED offers several professional credentials that align with different aspects of sustainability and building design. These certifications are categorized as follows:
LEED Green Associate
Description: The entry-level credential, designed for professionals who want to demonstrate their foundational knowledge of green building concepts.
Focus: Core concepts of LEED, sustainability practices, and environmental impact reduction.
Who It’s For: Anyone new to LEED, including students, professionals from related industries, or individuals interested in sustainability.
LEED Green Associate Exam Preparation Guide
LEED Accredited Professional (LEED AP)
Description: An advanced credential that demonstrates expertise in specific LEED rating systems.
Focus: Specialized knowledge in one or more LEED categories.
Specializations Available:
- Building Design and Construction (BD+C): For professionals involved in new construction or major renovations.
- Interior Design and Construction (ID+C): Focused on interior spaces and fit-outs.
- Operations and Maintenance (O+M): Targets professionals managing the efficiency of existing buildings.
- Neighborhood Development (ND): For planning and developing sustainable communities.
- Homes: Concentrates on single-family and multifamily residential projects.
Who It’s For: Professionals like architects, engineers, contractors, or consultants looking to specialize in a particular LEED system.
LEED Fellow
Description: The highest distinction offered by LEED, recognizing exceptional achievements and leadership in the green building field.
Eligibility: Reserved for seasoned professionals with at least 10 years of experience and significant contributions to the green building industry.
Who It’s For: Established leaders, educators, and innovators in sustainability.
In addition to broad certifications, individuals can pursue additional specialty designations or continuing education credits in areas like:
Net-zero energy and water design.
Resilience and adaptation strategies.
Renewable energy integration.
After certification, individuals are required to maintain their credentials through ongoing education. This ensures they stay updated with evolving sustainability standards and technologies.
Summary
LEED (Leadership in Energy and Environmental Design) is the world’s leading certification system for sustainable building practices, providing a framework for designing, constructing, and maintaining environmentally responsible spaces. For buildings, LEED certification promotes resource efficiency, energy savings, and healthier environments, with certification levels ranging from Certified to Platinum. The system applies to various building types and uses rating systems tailored to new constructions, interiors, existing buildings, neighborhoods, and homes.
For individuals, LEED credentials such as Green Associate and LEED AP demonstrate expertise in green building principles, offering enhanced career opportunities and industry recognition. Together, LEED-certified buildings and professionals drive the shift toward a more sustainable and resilient built environment, helping to mitigate environmental impact while fostering innovation and community well-being.